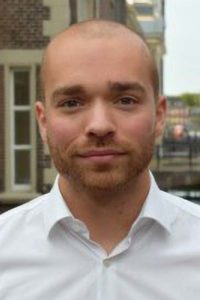
Michiel Zietse, MSc
Immune checkpoint inhibitors (ICIs) have revolutionized cancer treatment by offering durable responses to many patients with lung cancer. These monoclonal antibodies augment the T cell-mediated clearance of malignant cells by blocking the interaction between PD-1 and PD-L1, with or without CTLA-4 targeting.1
For an ever-increasing number of tumor types, ICIs continue to result in improved clinical outcomes, thereby rapidly extending the number of indications for which ICIs have become available as a treatment option. With promising combination strategies emerging, it can be expected that ICIs will remain the cornerstone of many cancer treatments in the future.
Unfortunately, these great advances in cancer treatment come at a price. It is anticipated that the use of ICIs will continue to rise with an annual growth rate of about 18%, resulting in a global total cost of $40 billion US in 2025.2 The combination of the cost of these drugs and the extended reimbursement puts considerable strain on healthcare budgets worldwide. Budgetary limitations may subsequently endanger patients’ access to these anticancer therapies, especially for those living in low- and middle-income countries.3
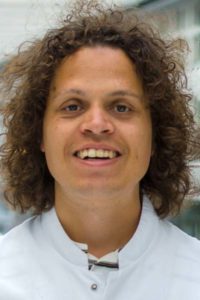
Ruben Malmberg, PharmD
To ensure the accessibility of ICI therapy, these drugs must be used in a more efficient manner. To that extent, healthcare professionals must collaborate to implement the most cost-effective dosing strategy of ICIs.
Accordingly, at Erasmus MC Cancer Institute, University Medical Center, Rotterdam, The Netherlands, we continuously seek new strategies to improve the cost-effectiveness of ICI therapy without compromising efficacy and patient safety. Previously, we have introduced interventions such as electronically rounding of doses, shortening of the infusion times, and vial sharing. These interventions were directly implementable and resulted in instantaneous cost savings and a reduction of drug spillage.3
Despite these initial actions, more far-reaching measures will likely be required to guarantee the accessibility of ICI therapy. Therefore, we propose dose-optimization tools as a practical solution to further reduce the costs of ICI treatment, with a particular focus on nivolumab and pembrolizumab.
Hybrid Dosing
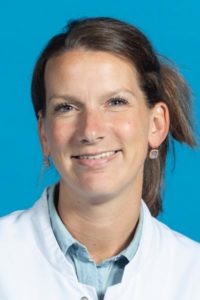
Daphne W. Dumoulin, MD
Nivolumab and pembrolizumab were initially registered with a recommended weight-based dosing (mg/kg) regimen, comparable to the established dosing strategies of classic anticancer agents.
This strategy is not very rational as monoclonal antibodies have distinct pharmacokinetic characteristics, such as slow linear drug clearance through proteolytic catabolism. Also, nivolumab and pembrolizumab show a small distribution volume (Vd). Their drug targets (T cells) are mainly located in the primary blood circulation, of which the volume only shows nonlinear correlation with body weight.4 Consequently, fixed dosing regimens were later adopted, as pharmacokinetic models confirmed the similarity of the dose distributions to weight-based dosing regimens for nivolumab and pembrolizumab.5,6
Although dosing was simplified by fixed dosing regimens, it also led to higher costs of ICI treatment. On average, patients receive a 10% and 22% higher ICI dose through fixed dosing compared to weight-based dosing for nivolumab and pembrolizumab, respectively.7
Therefore, we propose hybrid dosing, which combines weight-based dosing with the registered fixed-dose as a maximum dose (dose cap). This hybrid ICI dose leads to a sharp cost reduction, as shown in table 1. The cost-effectiveness of the hybrid dosing strategy can be further maximized through vial sharing and (electronic) rounding on whole ampoules within a ±10% margin. This approach minimizes the treatment costs and spillage without compromising the safety4 and efficacy of ICI treatment. When implemented on a global scale, this novel strategy has the potential to realize cost savings of billions of dollars.2
Pembrolizumab Strategies
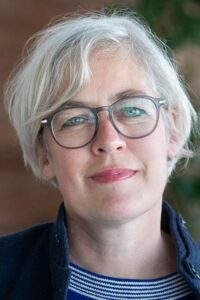
Anne-Marie C. Dingemans, MD, PhD
When pembrolizumab was first marketed, a dose of 2 mg/kg every 3 weeks (Q3W) was registered. Controversially, a fixed dose of 200 mg Q3W was later approved, estimated to increase treatment costs by 22% to 24% (or about $825 million yearly in the US) without further efficacy gains.7,8 According to weight-based dosing, the selected fixed dose is appropriate for patients weighing 100 kg. The average weight of real-life patients is more likely to be in the range of 75-80 kg, as was assumed in the dose-finding strategy of other ICIs.5 In this respect, selecting a fixed dose of 154 mg Q3W would have been more appropriate. Simulations by Freshwater et al. also support this assumption. They showed that fixed dose of 154 mg Q3W better matches the exposure parameters of the 2 mg/kg Q3W dosing regimen.6
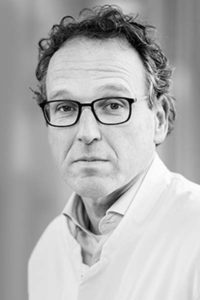
Joachim Aerts, MD, PhD
Attempts have been made to justify the selection of the 200 mg Q3W fixed dose by arguing that this dose ensures adequate target saturation in the intratumoral environment.9 However, the clinical relevance of intratumoral concentrations as a predictor of pembrolizumab efficacy remains uncertain. This statement also seems to undermine earlier remarks, where it was argued against dose adjustment based on the same principle.6 In an US Food and Drug Administration report, it has even been disclosed that peripheral target saturation is achieved at ≥ 0.1 mg/kg doses.10 This suggests that the dose can be even further reduced without compromising treatment efficacy, as dose-finding revolves around achieving target saturation.5
Currently, pembrolizumab is only available in vial sizes of 100 mg, thereby limiting the flexibility of a hybrid dosing regimen and rounding. To overcome this limitation, we implemented dose bands in our center corresponding to 100 mg for individuals ≤65 kg and 150 mg for individuals >65 kg (table 1).
For every 6-week extended dosing, we apply 100 mg dose bands of 200 mg, 300 mg, and 400 mg respectively (table 1). This has led to annual savings of approximately 22%.11 Additionally, the extended-dosing interval has reduced the patients’ time spent at the outpatient oncology unit, which is also a significant component of the total treatment costs.12
Nivolumab Strategies
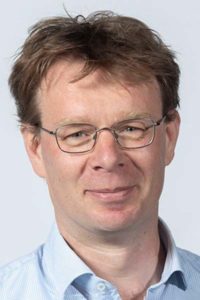
Stefan Sleijfer, MD, PhD
For nivolumab, several doses are currently approved across different indications. Initially, a 3 mg/kg every two weeks (Q2W) dose was registered. This was followed by subsequent approvals of the fixed doses of 240 mg Q2W and the extended interval of 480 mg every four weeks (Q4W) based on pharmacokinetic modeling.5,13 In addition, various doses of nivolumab in combination with ipilimumab are registered across several indications, including lung cancer.
Based on various studies, it can be assumed that nivolumab is currently dosed well above the minimum effective dose. No difference in PD-1 receptor occupancy was observed for dose levels ranging from 0.1-10 mg/kg Q2W during Phase 1 trials.14 Nevertheless, a dose of 3 mg/kg Q2W was selected for all monotherapy indications even though this dose results in trough concentrations that are 160 times higher than the half effective concentration (EC50) (0.1 μg/ml) after the first dose.15
More questions were raised during the registration of the nivolumab combination with ipilimumab for patients with melanoma. Here nivolumab was approved in a 1 mg/kg Q3W dosing regimen,5 even though ipilimumab induces clearance of nivolumab. Nonetheless, regulatory authorities concluded that the trough concentration was sufficient to ensure full target saturation and near maximum efficacy.16 In contrast, a dose of 360 mg Q3W in combination with ipilimumab 1 mg/kg Q6W was selected for various lung cancer indications.
This selection, however, was not based on superior response rates, as lower doses of nivolumab (1 mg/kg Q2W in combination with 1 mg/kg ipilimumab Q6W) resulted in comparable response rates during phase I testing.17 These findings support the hypothesis that the efficacy of nivolumab plateaus at lower doses than the currently registered doses.
No weight-based dose was registered for the extended-dosing intervals of nivolumab Q3W and Q4W. Given its similarity in the pharmacokinetic and pharmacodynamics properties to pembrolizumab, we have adopted proportional weight-based doses for the extended interval of nivolumab. Correspondingly, we use hybrid-dosing regimens of 3 mg/kg with a maximum dose of 240 mg Q2W, 4.5 mg/kg with a maximum dose of 360 mg Q3W, and 6 mg/kg with a maximum dose of 480 mg Q4W for nivolumab. Through hybrid dosing, we have managed to realize substantial savings of 10% for Q2W, Q3W, and Q4W strategies (table 1).7
Future directions
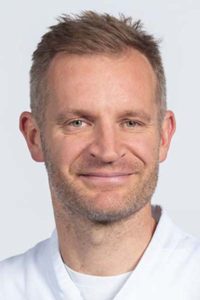
Roelof van Leeuwen, PharmD, PhD
It is anticipated that the use of ICIs will continue to grow, with their costs further stretching healthcare budgets globally and potentially limiting the accessibility of these highly effective treatments. Therefore, it is of the utmost importance to critically evaluate current dosing regimens of ICIs.
In combination with dose rounding, hybrid-dosing has proven a feasible strategy that immediately reduces the costs of ICI therapy without compromising the efficacy and patient safety. However, data suggests that the registered doses of both nivolumab and pembrolizumab are well above the minimum effective dose.9,10,14,15,16 Therefore, we strongly believe that the cost-effectiveness of ICI dosing can be further improved, by either dose reduction, extension of the dosing interval or by earlier discontinuation of ICI treatment in patients achieving a complete or partial response.18
Currently, numerous clinical trials are ongoing to investigate the efficacy and safety profiles of lower-dose ICI therapy.19,20,21 While we eagerly await new evidence for more cost-effective dosing strategies, we urge healthcare professionals to consider adopting and sharing hybrid dosing schedules for nivolumab and pembrolizumab to reduce the costs of ICI therapy without impacting the clinical outcomes.8
References
- 1. Robert C. A decade of immune-checkpoint inhibitors in cancer therapy. Nat Commun. 2020;11(1):3801.
- 2. The Business Research Company. Checkpoint Inhibitors Global Market Report 2021: COVID-19 Growth and Change to 2030
- 3. Patel A, et al. Improving access to immunotherapy in low- and middle-income countries. Ann Oncol. 2022.
- 4. Hendrikx J, et al. Fixed Dosing of Monoclonal Antibodies in Oncology. Oncologist. 2017;22(10):1212-21.
- 5. Zhao X, et al. Assessment of nivolumab benefit-risk profile of a 240-mg flat dose relative to a 3-mg/kg dosing regimen in patients with advanced tumors. Ann Oncol. 2017;28(8):2002-8.
- 6. Freshwater T, et al. Evaluation of dosing strategy for pembrolizumab for oncology indications. J Immunother Cancer. 2017;5:43.
- 7. Bayle A, et al. Switch to anti-programmed cell death protein 1 (anti-PD-1) fixed-dose regimen: What is the economic impact? Eur J Cancer. 2019;113:28-31.
- 8. Goldstein DA, et al. A Phamacoeconomic Analysis of Personalized Dosing vs Fixed Dosing of Pembrolizumab in Firstline PD-L1-Positive Non-Small Cell Lung Cancer. J Natl Cancer Inst. 2017;109(11).
- 9. Li TR, et al. Pivotal Dose of Pembrolizumab: A Dose-Finding Strategy for Immuno-Oncology. Clin Pharmacol Ther. 2021;110(1):200-9.
- 10. FDA. Review Memo: Supplemental Biologics License Application for Pembrolizumab 400 mg every six weeks 2020.
- 11. Diekstra MHM, et al. Letter to the editor: Comments on ‘A six-weekly dosing schedule for pembrolizumab in patients with cancer based on evaluation using modelling and simulation’. Eur J Cancer. 2020;138:54-6.
- 12. Tan SS, et al. The unit costs of inpatient hospital days, outpatient visits, and daycare treatments in the fields of oncology and hematology. Value Health. 2010;13(6):712-9.
- 13. Zhao X, et al. Model-based evaluation of the efficacy and safety of nivolumab once every 4 weeks across multiple tumor types. Ann Oncol. 2020;31(2):302-9.
- 14. Topalian SL, et al. Safety, activity, and immune correlates of anti-PD-1 antibody in cancer. N Engl J Med. 2012;366(26):2443-54.
- 15. FDA Clinical Pharmacology and Biopharmaceutics Review(s) Application number: 125554Orig1s0002014.
- 16. EMA. Assessment report Opdivo Procedure No. EMEA/H/C/003985/II/0003.
- 17. Hellmann MD, et al. Nivolumab plus ipilimumab as first-line treatment for advanced non-small-cell lung cancer (CheckMate 012): results of an open-label, phase 1, multicohort study. Lancet Oncol. 2017;18(1):31-41.
- 18. Netherlands Trial Register. Trial NL7293 (NTR7502).
- 19. ClinicalTrials.gov. National Library of Medicine (US). Identifier NCT04295863.
- 20. EU Clinical Trials Register. European Medicines Agency. EudraCT 2021-001707-32.
- 21. ClinicalTrials.gov. National Library of Medicine (US). Identifier NCT04909684.